The development of commercially available malaria diagnostic tests and their extent of application in the Philippines: a systematic review
Highlight box
Key findings
• Despite the technological advances in the commercially available malaria diagnostic tests, the diagnostic tests applied in the Philippines still rely mostly on microscopy and rapid diagnostic tests (RDTs). Regardless, the country has recorded a significant decrease in the number of confirmed cases and reported deaths from malaria since the year 2000.
What is known and what is new?
• Prevalence and transmission have been significantly reduced by prioritizing prophylaxis, drug treatment, and prevention of infection.
• Diagnostic practice using microscopy and RDTs that were used to confirm cases may not provide an accurate picture of malaria incidence, as this might neglect residual malaria transmission.
What is the implication, and what should change now?
• To verify a successful malaria elimination program, technologically advanced and commercially available malaria diagnostic tests should be used for the detection of malaria resurgence and the assessment of the epidemiological situation in a country gearing towards malaria elimination.
Introduction
Background
The movement to eliminate malaria has resulted in a decrease in morbidity and mortality worldwide (1). However, malaria remains a global health problem, with an estimated 241 million cases and 627,000 malaria deaths worldwide in 2020. This represents about 14 million more cases and 69,000 more deaths in 2020 when compared to 2019 (2). In addition, in non-endemic regions such as the Middle East and Europe, cases of imported malaria persist (3). In the case of the Philippines, following progress in malaria control, there were still provinces where malaria transmission exists (e.g., Palawan, Sarangani, and South Cotabato), as well as imported malaria that is continuously increasing (2,4,5).
Rationale and knowledge gap
Therefore, there is a need for a sensitive diagnostic approach that can identify residual malaria transmission. A diagnostic test that would allow early detection in asymptomatic individuals with low parasitemia. However, routine diagnostic tests like microscopy and rapid diagnostic tests (RDTs) may not detect residual malaria transmission. Thus, there is a need for the adoption of a diagnostic test that is useful in a country that is geared towards malaria elimination.
Objective
This review paper attempts to summate published literature related to the development of malaria diagnostic tests. It focuses on malaria biomarkers [e.g., Plasmodium falciparum histidine-rich protein (PfHRP2), parasite lactate dehydrogenase (pLDH), etc.] or targets (e.g., DNA or ssRNA) when they were first identified to diagnose malaria, the development of the methods that eased the detection of these biomarkers or targets, their commercialization in the form of commercial RDTs or nucleic acid amplification tests (NAATs), and their resulting clinical trial or randomized control trial for determination of their diagnostic specificity and sensitivity. This study also aims to associate these developments in malaria diagnostic tests with the malaria detection methods done in the Philippines and their effects on the country’s malaria mortality and morbidity rates using the data derived from the World Malaria Report 2010–2021 (1,2,6-15). We present this article in accordance with the PRISMA reporting checklist (available at https://jphe.amegroups.com/article/view/10.21037/jphe-23-46/rc).
Methods
Search strategy
Studies related to malaria biomarkers or targets and the development of its detection methods until December 2022 were systematically searched in Medline through PubMed. The search term used to find potentially relevant studies was “malaria diagnosis”. In PubMed, the authors ticked the article types “clinical trial” and “randomized control trial” only. The authors also explored the references in the selected articles to search for the earliest published research that studied the detection of a malaria biomarker or target in clinical samples.
The eligibility criteria and study selections
The inclusion criteria for selection in the present study were that any included studies must be primary studies, such as research articles and short communications about clinical trials or randomized controlled trials related to malaria biomarkers or targets and the development of the methods for their detection or quantification in clinical samples. The exclusion criteria were (I) studies without data of interest; (II) studies without full text; and (III) review articles, conference information and abstracts, correspondence, news, editorials, and mini reviews and discussions. The authors (Palmares AJ and Martin I G) screened the studies according to the eligibility criteria for potentially relevant studies.
Data extraction
The data of each study were initially extracted as follows: author name, year of publication, country, setting (population characteristics in terms of symptoms), sample size, device or method used, target analyte, gold standard, and their sensitivity and specificity versus the gold standard. All data extraction was performed by the authors. After data extraction, the data were grouped into five categories according to biomarkers and targets (regardless of diagnostic formats), such as the detection of malaria parasites in red blood cells (through microscopy or light scatter), PfHRP-2, pLDH, hemozoin, and DNA/ssRNA genes [through DNA hybridization, polymerase chain reaction (PCR), and loop-mediated isothermal amplification (LAMP)] in blood samples.
Results
Search results
The initial search yielded 1,786 records from PubMed (Figure 1). Out of those records, 10 of the duplicates were removed. After screening the titles and abstracts of the remaining 1,776 records, 1,689 records were excluded. All the reports (articles) from the remaining 87 records were retrieved. Following the assessment of the reports for eligibility, an additional 59 reports were excluded for reasons such as the studies were about the development of diagnostic algorithms, the economic impact of microscopy and RDTs in treatment, behavioral studies on the use of RDTs and the practice of microscopy, and unspecified biomarkers used for the determination of sensitivity and specificity. Using the 28 reports included from PubMed, an additional 13 reports from citation searching were retrieved, were found eligible, and were included in the final list of 41 studies. Out of the 41 studies selected, 5 studies reported the detection of malaria-infected red blood cells (RBCs) via microscopy [using acridine orange and quantitative buffy coat (QBC)] or light scatter (16-20), 10 studies reported the detection of PfHRP-2 only (21-30), 4 studies reported the detection of pLDH only (31-34), 1 study reported the detection of both PfHRP-2 and pLDH (35), 6 studies reported the detection of hemozoin (36-41), and 15 studies reported the detection of DNA/ssRNA genes of which 2 were via DNA hybridization techniques (42,43) and 13 were via NAATs, of which 8 were PCR techniques (44-51), and 5 were LAMP techniques (52-56).
Characteristics of the included studies
The characteristics of the included studies are shown from Tables 1-5. Among the 41 studies, only two related studies were conducted in the Philippines, one each by an international (17) and a local researcher (35). The earliest study reporting the detection of malaria via microscopy using QBC and acridine orange was in 1988 and 1991, respectively (16,18). Other biomarkers/targets such as hemozoin, DNA, PfHRP-2, and pLDH were first reported in 1983, 1984, 1991, and 1993, respectively (21,31,36,42). Detection of malaria DNA via hybridization technique, PCR, and LAMP was first published in 1984, 1990, and 2005, respectively (42,44,52) (Figure 2).
Table 1
Study | Country | Setting (population characteristics) | Sample size | Device/method | Gold standard | Sensitivity (%) | Specificity (%) |
---|---|---|---|---|---|---|---|
Ref. (16) | Ethiopia | Population based (symptomatic) | NM | Fluorescence microscopy (QBC) | Microscopy | NM | NM |
Ref. (17) | Philippines | Population and hospital based (villagers and patients, symptomatic and asymptomatic) | 588 | Fluorescence microscopy (QBC) | Microscopy | 70–96 | 93 |
Ref. (18) | Japan | NM | NM | Fluorescence microscopy (acridine orange) | NM | NM | NM |
Ref. (19) | France | Population based (travelers returning form malaria endemic areas, asymptomatic) | 243 | Fluorescence microscopy (acridine orange) | Microscopy | 96 | 95 |
Ref. (20) | Burkina Faso | Population (symptomatic) | 908 | XN-30 hematology analyzer (light scatter) | Microscopy | 71 | 100 |
RBCs, red blood cells; Ref., reference; NM, not mentioned; QBC, quantitative buffy coat.
Table 2
Study | Country | Setting (population characteristics) | Sample size | Device/method | Gold standard | Sensitivity (%) | Specificity (%) |
---|---|---|---|---|---|---|---|
Ref. (21) | Colombia | Population based (adult, symptomatic) | 54 | Western blot and dot blot (plasma) | Case definition by symptoms | 95–98 | 100 |
Ref. (22) | Tanzania | Population based (symptomatic) | 138 | ParaSight®-F test | Microscopy | 89 | 88 |
Ref. (23) | Thailand | Population based (children and adults, symptomatic) | 913 | ParaSight® TM-F Dipstick | Microscopy | 93 | 98 |
Ref. (24) | Senegal | Population based (children and adults, symptomatic) | 66 | ParaSight® TM-F Dipstick, ICT Malaria P.f. | Microscopy | 86, 89 | 93, 100 |
Ref. (25) | Bangladesh | Hospital based (adult, symptomatic) | 59 | ParaSight® TM-F Dipstick t | Microscopy | 97 | NM |
Ref. (26) | Tanzania | Population based (symptomatic) | 1,180 | Paracheck-pf | Microscopy | 95 | 96 |
Ref. (27) | Kenya | Population based (children, symptomatic) | 515 | Vision Biotech | Microscopy | 81 | 79 |
Ref. (28) | Nigeria | Population based (children, symptomatic) | 1,860 | SD Bioline Ag-Pf | Microscopy | 93–94 | 41–52 |
Ref. (29) | Burkina Faso | Population based (pregnant women, asymptomatic) | 900 | SD Bioline Ag-Pf | Microscopy, PCR | 92, 76 | 82, 97 |
Ref. (30) | Ghana | Population based (pregnant women, asymptomatic) | 2,071 | CareStart HRP-2, RDT | PCR | 98 | 99 |
Ref. (35) | Philippines | Population based | 519 | Paracheck Pf, ParaHIT f | Microscopy | 5–12, 10–67 | 91–100, 83–95 |
PfHRP2, Plasmodium falciparum histidine-rich protein; Ref., reference; NM, not mentioned; RDT, rapid diagnostic test; PCR, polymerase chain reaction.
Table 3
Study | Country | Setting (population characteristics) | Sample size | Device/method | Gold standard | Sensitivity (%) | Specificity (%) |
---|---|---|---|---|---|---|---|
Ref. (31) | NM | NM | NM | Photometry | NM | NM | NM |
Ref. (32) | Germany | Population based (travelers from endemic areas, symptomatic) | 121 | Photometry | Microscopy | 76 | 97 |
Ref. (33) | South Africa | Population based (children and adult, symptomatic) | 122 | OptiMAL | Microscopy, PCR | NM | 90 |
Ref. (34) | Papua New Guinea | Population based (symptomatic) | 255 | OptiMAL | Microscopy | 39–68 | 59–92 |
Ref. (35) | Philippines | Population based | 519 | OptiMAL | Microscopy | 11–97 | 18–92 |
pLDH, parasite lactate dehydrogenase; Ref., reference; NM, not mentioned; PCR, polymerase chain reaction.
Table 4
Study | Country | Setting (population characteristics) | Sample size | Device/method | Gold standard | Sensitivity (%) | Specificity (%) |
---|---|---|---|---|---|---|---|
Ref. (36) | Saudi Arabia | Population based | NM | Dark field microscopy | Microscopy | NM | NM |
Ref. (37) | Thailand | Population based (symptomatic) | 23 | Dark field microscopy with Imaging algorithm | RDT, Microscopy and PCR | 95 | 97 |
Ref. (38) | South Africa | Population based (symptomatic) | 852 | Cell-Dyn CD4000 depolarization analysis | Microscopy and PCR | 80 | 83 |
Ref. (39) | Nigeria | Population based (children, travelers from endemic areas, symptomatic) | 13 | Magneto-Optic Device | RDT | 100 | 100 |
Ref. (40) | Kenya | NM | NM | LED microscope with Imaging algorithm | Microscopy | NM | NM |
Ref. (41) | Brazil | Population based | 276 | Magneto-Optic Device (Gazelle™) | Microscopy and PCR | 72–96 | 99–100 |
Ref., reference; NM, not mentioned; RDT, rapid diagnostic test; LED, light-emitting diode; PCR, polymerase chain reaction.
Table 5
Study | Country | Setting (population characteristics) | Sample size | Device/method (target) | Gold standard | Sensitivity (%) | Specificity (%) |
---|---|---|---|---|---|---|---|
Ref. (42) | Colombia, Tanzania, Kenya | Hospital and population based (travelers from endemic areas, symptomatic) | 35 | Probe hybridization assay, dot blot hybridization (DNA) | Microscopy | 97 | NM |
Ref. (43) | Kenya | Population based (women only, symptomatic) | 24 | Probe hybridization assay dot blot hybridization (DNA) | Microscopy | 83 | NM |
Ref. (44) | France | Population based (symptomatic) | NM | Conventional PCR (DNA) | Microscopy | NM | NM |
Ref. (45) | Thailand | Laboratory | NM | PCR with probe hybridization (DNA) | NM | NM | NM |
Ref. (46) | Thailand | Population based (migrant miners, symptomatic) | 196 | Conventional PCR (ssRNA) | Microscopy | 82 | 80 |
Ref. (47) | Thailand | Population based | 25 | Nested RT-PCR (ssRNA) | NM | NM | NM |
Ref. (48) | Thailand | Population based (asymptomatic) | 300 | PCR-ELISA, microtiter plate hybridization assay (DNA) | Microscopy | 91 | 96 |
Ref. (49) | Zambia | Population based (symptomatic) | NM | Photo-induced electron transfer-PCR (ssRNA) | Microscopy | 29 | 99 |
Ref. (50) | Oman | Population based (adult, asymptomatic) | NM | qRT-PCR (early-stage P. falciparum gametocytes genes | NM | NM | NM |
Ref. (51) | Malaysia | Population based (symptomatic) | 134 | QuantiFastTM, abTESTM | Microscopy | 98, 100 | 99, 99 |
Ref. (52) | Thailand | Population (symptomatic) | 202 | LAMP (DNA) | PCR | 95 | 99 |
Ref. (53) | Thailand | Population based (children and adults, symptomatic) | 111 | LAMP (DNA) | Microscopy | 99 | NM |
Ref. (54) | Senegal | Population based (children and adults, symptomatic) | 216 | Illumigene Malaria LAMP assay (DNA) | PET PCR | 97 | 88 |
Ref. (55) | Ethiopia | Population based (pregnant women, symptomatic) | 87 | Loopamp™ malaria Pan/Pf detection kits (DNA) | Nested PCR | 100 | 94 |
Ref. (56) | Cameroon | Population based (symptomatic neonates, asymptomatic adults) | 279 | Reverse transcription - LAMP (DNA) | Microscopy and RDT | 75–76 | NM |
Ref., reference; NM, not mentioned; PCR, polymerase chain reaction; LAMP, loop-mediated isothermal amplification; RT-PCR, reverse transcription-PCR; PET-PCR, photoinduced electron transfer PCR; ELISA, enzyme linked immunosorbent assay; RDT, rapid diagnostic test.
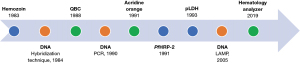
Scope of applied diagnostic test for malaria worldwide
The most common equipment used for the diagnosis of malaria infection was brightfield, darkfield, and/or fluorescence microscopes to detect gametocytes, trophozoites, schizonts, or hemozoin in Giemsa or acridine orange-stained blood smears and QBC preparations (Table 1). Furthermore, the detection of malaria biomarkers such as PfHRP2 and pLDH using immunochromatography lateral flow devices or RDTs (Tables 2,3), hemozoin by magneto-optical devices (Table 4), and detection of Plasmodium sp. DNA/ssRNA (Table 5) by PCR and LAMP, were also widely used and extensively reviewed elsewhere (57-65). Among these methods, identifying malaria parasites in Giemsa-stained blood smears and PfHRP2 (in whole blood) with RDTs was the most common, especially in resource-poor countries (1,2). Currently, many candidate malaria proteins are being studied as potential biomarkers for the next-generation malaria RDTs (66). Image analysis software for microscopy was also developed with features of image recognition and machine learning algorithms to identify and quantify parasitemia in blood smears (67,68). In addition, lab-on-a-chip diagnostic approaches have been continually enhanced for protein, nucleic acid, and cell-based detection of malaria (69). There were many advances, but they were not yet available on the market, or widely adopted for malaria diagnosis, especially for the field diagnosis of malaria in the Philippines.
Malaria diagnosis by detection of Plasmodium parasite within RBCs
The World Health Organization (WHO) has recommended microscopy as one of the diagnostic tests for suspected malaria cases (8). In the Philippines, malaria diagnosis by microscopy was adopted in 2003 and has been implemented with supporting data since 2010 as part of the WHO intervention policies and strategies (Table 6) (1,2,6-15). In most studies listed in Tables 1-5, the gold standard (reference method) for malaria diagnosis was microscopy of thin and thick blood smears using Giemsa stain, whether it was for the study of malaria PfHRP2, pLDH, hemozoin, or DNA/ssRNA (34 out of 41 studies). Microscopy remains the most common gold standard used, as it is highly available (even in a remote laboratory setting) and is easily used and standardized. An improvement in sample preparation for malaria microscopy, such as the QBC tube apparatus, uses microhematocrit centrifugation to concentrate Plasmodium spp. into the top of the erythrocyte column for direct examination of a capillary or venous blood sample. Tubes are mostly pre-coated with acridine orange to provide a stain that induces fluorescence in Plasmodium spp. when viewed using a fluorescence microscope (16-19). However, this method was not stated as a recommended diagnostic test for suspected malaria cases due to the need for a fluorescence microscope. Currently, an automated hematology analyzer that combines standard hemocytometry and software analysis to detect malaria-infected RBC (by light scatter) is available on the market. These advances can be a useful tool for mass screening in malaria control programs, as they can be routinely done along with a complete blood count (20). In the Philippines, there seem to be no studies about the development of methods for the detection of Plasmodium parasites within RBCs. Regardless, the country still relies on routine tests for malaria diagnosis, such as the use of traditional bright-field microscopy of thin and thick blood smears (1,2,6-15).
Table 6
Policies/strategies | Year adopted | Actually implemented | No supportive data reported |
---|---|---|---|
Patients of all ages should receive diagnostic test | 2004 | 2010 to 2017 | 2018 to 2020 |
Malaria diagnosis (microscopy & RDT) is free of charge in the public sector | 2003 | 2010 to 2017; 2019 to 2020 | 2018 |
RDTs used at community level | 2002 | 2010 to 2020 | – |
G6PD test is recommended before treatment with primaquine | 2011 | 2013 to 2017 | 2018 to 2020 |
RDT, rapid diagnostic test; G6PD, glucose-6-phosphate dehydrogenase deficiency.
Malaria diagnosis by detection of PfHRP-2, pLDH, and hemozoin
The WHO has also recommended the use of rapid RDT (along with microscopy) as one of the diagnostic tests for suspected malaria cases worldwide (8). In the Philippines, malaria diagnosis by RDT at the community level was adopted in 2002. RDT use was implemented (with supporting data since 2010) as part of the WHO intervention policies and strategies (Table 6) (1,2,6-15). A detailed discussion of RDT’s principles, advantages, and disadvantages has been discussed extensively elsewhere (58,62,70). The common biomarkers detected by RDTs are PfHRP-2 and pLDH. Other biomarkers, such as hemozoin, are usually detected through their optical and magnetic properties using polarized laser light (38,41,62). Among all the included studies, only two have used PfHRP-2 RDT as the gold standard, usually along with microscopy and/or PCR (39,56). Regarding the first reports on these biomarkers, the first article that describes PfHRP-2 for the diagnosis of malaria using blood samples was published in 1991 by Parra et al. Since P. falciparum in infected RBCs synthesizes HRP-2 as a water-soluble protein into the circulation, this biomarker was first detected in blood samples using western blot and dot blot techniques (21). However, one of the emerging concerns for malaria diagnosis is the worldwide reports of PfHRP2 gene deletions that led to false negative results. This may affect malaria control and elimination efforts in the Philippines (71). Regarding the other biomarker, the first report that describes pLDH for the determination of P. falciparum levels in whole blood hemolysates and plasma of malaria patients was published in 1993 by Makler and Hinrichs (31). In this assay, pLDH activity was measured in a reaction leading to the reduction of a coupling indicator dye to a colored product, whose absorbance was measured by photometry (31). Finally, the detection of hemozoin (malaria pigment) was first reported in 1983 by Jamjoom. Hemozoin is a heme crystal produced by Plasmodium spp. when it digests hemoglobin (36). It has a metal-ion-containing prosthetic group that appears brighter when illuminated in a parasite-infected cell under a dark-field microscope (40). Eventually, these labor-intensive and time-consuming methods like western blot and photometry for detection of PfHRP-2 and pLDH have both evolved into rapid immunochromatographic (lateral flow) devices (70), and hemozoin’s detection by dark-field microscopy was automated using computer-mediated imaging algorithms, as well as commercialized using automated magneto-optical devices (64). Regarding the studies of these biomarkers in the Philippines, they were mostly related to the evaluation of RDTs for the diagnosis of P. falciparum and non-P. falciparum infections. They focused mainly on the comparison of methods, such as comparing a whole blood RDT to a saliva enzyme-linked immunosorbent assay (ELISA) (72), acceptance of RDTs by community health workers (73), comparing RDT blood collection and transfer methods (74), and comparing various RDT brands (75). One study investigated factors that may lead to false results in RDTs (76). According to their collective findings, RDT reliance for malaria diagnosis is subject to mostly operator errors (74,77).
Malaria diagnosis by detection of DNA/ssRNA
Currently, the WHO has not yet listed NAATs such as PCR or LAMP as one of the diagnostic tests for clinical management and in the routine surveillance systems of malaria worldwide (1,8). It is a fact that these techniques are challenging to use in low-resource settings; therefore, they are generally not available for large-scale field use in malaria-endemic areas. Nonetheless, a detailed discussion of malaria diagnosis by detection of DNA or ssRNA, its principles, developments, advantages, and disadvantages, has been described extensively elsewhere (57,64,78). Regarding the first article that describes the detection of malaria DNA for the diagnosis of malaria using blood samples, it was published in 1984 by Franzén et al., using probe hybridization and dot blot hybridization assays (42). Eventually, NAATs for malaria diagnosis were developed, such as in the form of a conventional PCR by Jaureguiberry et al. in 1990 (44), and in the form of LAMP by Poon, Wong, and Ma in 2005 (52).
In the Philippines, there seem to be no studies about the development of NAATs for malaria, though they have been used for prevalence studies from 2010 to 2013 in certain towns in Bataan, Occidental Mindoro, and Palawan provinces (4). In the study, no PCR-confirmed infections were found in Bataan and Occidental Mindoro, but in Palawan, PCR detected more malaria infections (36.7% and 38% higher) than microscopy and RDT, respectively (4). PCR has also been used for prevalence studies of malaria in selected provinces of Mindanao during the same period. Malaria prevalence by PCR was 3.8%, 10%, and 4.2% in Sarangani, South Cotabato, and Tawi-Tawi, respectively (5). The studies used a conventional PCR approach, for which the PCR primers were first described in 1993 (46). Lastly, in eight of the 41 included studies, PCR was used as the gold standard for malaria diagnosis. Two for the study of PfHRP-2 and pLDH RDTs, three for hemozoin detection, and three for the development of LAMP assays.
Sensitivity and specificity of conventional diagnostic tests
Regarding the performance of RDTs (with microscopy as the gold standard) in symptomatic patients, it was stated that some commercial RDTs that detect PfHRP2 (e.g., ParaSight®, Becton Dickinson, New York, USA; SD Bioline, Abbott Diagnostics Korea Inc., Seoul, South Korea, etc.) have a sensitivity ranging from 81% to 94% and a specificity from 41% to 100% (22-28) (Table 2). In addition, some RDTs that detect pLDH (e.g., optimal) have a sensitivity ranging from 39% to 68% and a specificity from 59% to 92% (33,34) (Table 3). Lastly, some commercial devices that detect hemozoin (e.g., Cell-Dyn, Abbott Diagnostics, Chicago, USA and Gazelle™, Hemex Health, Portland, USA) have a sensitivity ranging from 72% to 96% and a specificity from 83% to 100% (38,41) (Table 4). However, the performance of these methods was not determined in a study that included asymptomatic participants who lived in malaria endemic areas. In contrast, field surveys using RDTs among asymptomatic participants in malaria endemic areas produce more varied results. RDTs that detect PfHRP2 (e.g., ParaCheck Pf, Orchid Biomedical Systems, Goa, India and ParaHIT f, Span Diagnostics, Gujarat, India) had a sensitivity ranging from 5% to 67% and a specificity from 83% to 100%, while RDTs that detect pLDH (e.g., OptiMAL, Diamed AG, Cressier, Switzerland) had a sensitivity ranging from 11% to 97% and a specificity from 18% to 92% (35). With all these variable performances in the field, malaria case identification, their management, and overall efforts on control and elimination can get compromised. Possible factors that caused this variability are adverse environmental conditions (e.g., humidity, temperature, etc.) that led to the deterioration of equipment (e.g., test strips) or random errors caused by the personnel performing the procedures (35). Regarding the performance of RDTs that detect PfHRP2 (with a real-time PCR as the gold standard) in asymptomatic participants, it had a sensitivity of 76% and a specificity of 97% (29) (Table 2). A similar study, with a conventional (qualitative) PCR as the gold standard had a sensitivity of 98% and a specificity of 99% (30). Nonetheless, the RDTs used in these studies can potentially produce false negative results from PfHRP2 gene deletion, leading to undiagnosed infections with P. falciparum (71,79). Therefore, a more advanced commercial method that can detect malaria DNA or ssRNA (e.g., PCR, LAMP) in blood samples regardless of mutations could improve the overall diagnostic performance.
Sensitivity and specificity of DNA/ssRNA based tests
Regarding the summary of studies on the performance of DNA/ssRNA-based tests (with microscopy as the gold standard) on symptomatic patients, one study stated that the sensitivity and specificity of commercial PCR methods (e.g., QuantiFast™, Qiagen, Hilden, Germany and abTES™, AITbiotech, Singapore, Singapore) had an average of 99% (51). In another study, commercial LAMP methods (e.g., Illumigene®, Meridian Bioscience Inc., Cincinnati, USA and Loopamp™, Eiken Chemicals, Tokyo, Japan) with conventional PCR as the gold standard had sensitivity and specificity averaging at 97% and 91%, respectively (55,56). However, these studies did not include asymptomatic participants. Nonetheless, in the study of Reyes et al., a conventional PCR detected more malaria infections (36.7% and 38% higher) than microscopy and RDTs, respectively (4). In addition to their greater sensitivity, PCR-based tests have the potential to determine parasite load, species, and drug resistance. LAMP, in contrast, though it requires less equipment, it cannot determine for parasite load and mutations since its qualitative (70). Overall, these NAATs may have higher detection rates for asymptomatic malaria with residual parasitemia, and they could be more useful in countries gearing towards malaria elimination. However, they are also subject to variability in sample preparation, the amplification step, and the read-out of the results; thus, their variants (e.g., real-time, multiplex, nested, etc.) may also have varying sensitivity and specificity (70).
Financing malaria control
Regarding the trends in the Philippines domestic financing and international disbursement in relation to meeting global malaria control targets, funding has been decreasing in the past decade. The Global Fund is the single largest source of funding for malaria, accounting for 60.4% of total disbursed funding from 2010 to 2019. The funds for malaria control from the Global Fund and other non-government contributions decreased from US$ 22.6 million in 2010 to US$ 3.4 million in 2019. Available information suggests that domestic funding is generally less than US$0.5, with an average of US$0.23 per person at risk from 2010 to 2020 (1,2,6-15). Regarding the financing of malaria diagnostic tests, though cost is an important factor when suggesting a preferable methods that may fill existing gaps in asymptomatic malaria detection, this factor was not included in the review as additional data would be required to estimate the costs per assay, including maintenance of facilities, equipment, and reagents in the Philippines. Regardless, lower numbers of reported malaria cases may have led to the reductions in funding. Therefore, the implementation of newer technology on a national or regional scale may be hindered by inadequate financial support.
Status of malaria diagnosis in the Philippines
The Philippines has adopted the policies recommended by the WHO for universal parasitological testing of suspected malaria (Table 6) (1,2,6-15). The current method of applied parasitological confirmation of patients with suspected malaria is brightfield microscopy of Giemsa-stained thick and thin blood smears and RDTs. Since RDT is the only commercially available test feasible in the field setting, this could be the reason why research on malaria diagnostic tests focused mostly on the proper implementation of RDT use in the country (35).
Regarding the extent to which the Philippines has adopted the policies for universal diagnostic testing of suspected malaria cases, the reported status is shown in Figure 3 (1,2,6-15). On average, the reported malaria cases by species in the Philippines from 2010 to 2020 (Figure 3A) were mostly P. falciparum (87.7%) and P. vivax (18.1%). The number of total reported cases decreased from 2010 to 2020 (Figure 3B). However, there was an increase in imported malaria cases, from 68 cases in 2014 to 95 in 2019, but the reported cases were down to 26 in 2020 due to restrictions on international travel brought on by the coronavirus disease 2019 (COVID-19) pandemic (Figure 3B). Regarding microscopic examination (Figure 3C), the number of patients tested decreased from 2013 to 2020. Meanwhile, there was an increasing trend in the use of RDTs from 2011 to 2018. However, there was an abrupt drop in reported RDT use in 2019 and 2020. Nonetheless, the percentage of treated cases who received a prior parasitological test was almost 100% from 2010 to 2019. Regarding the slide positivity rate (Figure 3D), there seems to be a decreasing trend (6.2% in 2010 to only 0.8% in 2019). Lastly, the annual blood examination rate (ABER), which refers to the number of parasitological tests (by microscopy or RDT) undertaken per 100 people at risk per year (expressed as a percentage) was on average of 0.6% (1,2,6-15). The estimated number of malaria cases per 1,000 persons at risk of malaria (Figure 4A) shows a reduction in case incidence, with the highest incidence of 3.5% in 2004 and the lowest incidence of 0.2% in 2018. Starting in 2010, the case incidence rate was less than 1 per 1,000 people at risk. There was an estimated average of 275 malaria deaths annually between 2000 and 2009 but between 2010 and 2020, the estimated average was down to 56 (Figure 4B). The reported numbers of malaria cases and deaths are used as core indicators for tracking the progress of malaria control programs. Based on the case and death reports recorded continuously over time, it went from 536 deaths in the year 2000 to only 3 deaths in the year 2020 (1,2,6-15).
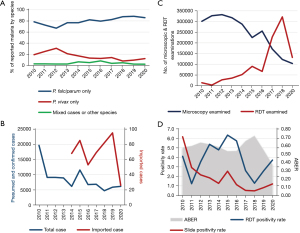
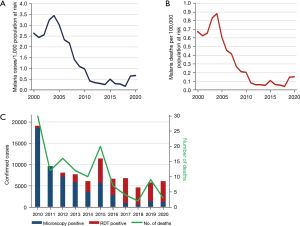
Discussion
Key findings
Despite there being no changes in the diagnostic policies and the very low ABER (0.6%), the burden that malaria places on the health system of the country is continually decreasing, based on the data retrieved from the WHO World Malaria Reports from 2010 to 2020. Aside from conventional diagnostic tests (microscopy and RDTs), government interventions such as community control programs, vector control programs, and anti-malarial drug interventions may have significantly contributed to the declining number of deaths from malaria in the Philippines, to the extent that the number of malaria deaths reported was near zero (2 reported deaths) in 2018. Nonetheless, the RDTs used by the National Malaria Control and Elimination Program (NMCEP) for identifying malaria cases may potentially produce false negative results, due to emerging mutations of target biomarkers (e.g., PfHRP-2) leading to undiagnosed P. falciparum infections.
Strengths and limitations
To the best of our knowledge, this study is the first review that assesses the contribution of the Philippines in the discovery of malaria biomarkers or targets, their development and commercialization as diagnostic tests, their extent of application in the country, and their effects on the country’s malaria mortality and morbidity rates. This type of information is significant since a specific and sensitive diagnostic test is important in identifying asymptomatic cases with light parasitemia. In this review, some limitations were identified. First, this review was done using a single medical database, such as PubMed. This was the only database selected, as it also contains the medical journals indexed in Scopus, Science Direct, and Wiley, but with an emphasis on research regarding diagnostic tests that have undergone clinical trials and randomized control trials. Related literature obtained from this search may not be exhaustive; therefore, a search in other health science-focused databases that do not overlap with PubMed can be done to gather more information. Second, mortality and morbidity rates (e.g., estimates of case incidence rates, confirmed malaria cases, reported deaths, etc.) and diagnostic policies were acquired from data published by the WHO World Malaria Reports from 2010 until 2021; therefore, the most current trends in mortality and morbidity rates are not recent and may sharply change in just one year at the provincial and national level. Other information, such as the frequency of malaria species identified, the number of microscopic and RDT examinations done, the malaria positivity rate, and ABER, was also obtained from the WHO World Malaria Reports. Lastly, no local data (municipal, city, or provincial level) compiled by the Philippines Department of Health’s (DOH) National Malaria Control and Elimination Program was included since the online published data were lacking and not updated. Therefore, more local data (published or unpublished) may need to be sought from the national, regional, or provincial government health agencies.
Comparison with similar researches
Several review articles focus on the enumeration of malaria diagnostic tests (e.g., ELISA, PCR, flow cytometry, etc.) and their advancements. Krampa et al. and Fitri et al. furthermore cited each of their advantages, disadvantages, and prospective uses (64,70). This led to recommendations such as the development of more technologically advanced devices such as biosensors coupled to flow cytometers with multiplex and quantitative ability (64). Other reviews by Jain et al. and Yerlikaya et al. focus on the use of proteomic databases to identify novel diagnostic biomarkers (62,66). This revealed that every malaria biomarker has some limitations and may need an in-depth understanding of its metabolism and physiology to determine its best use. On the other hand, this current review is different as it seeks to explain why, despite the common use of microscopy and RDTs alone, the trend of mortality and morbidity rates seems to be decreasing and to rationalize why there’s a need for more advanced and commercially available diagnostic tests.
Explanations of findings
The Philippines might have prioritized prevention of infection, case management, and drug treatment; therefore, the problem of morbidity and mortality rates was circumvented despite the country focusing only on microscopy and RDTs for identifying cases of malaria. Specifically, the prevention was through the use of long-lasting insecticidal nets (LLINs) and indoor residual spraying (IRS). The LLIN can shield the person under the net against mosquito bites, and the IRS can shorten the lifespan of mosquitoes, especially the adult female Anopheles spp. Usually, where IRS is conducted, LLINs are also applied (1,10). When it comes to the LLIN policy and implementation, LLINs are distributed free of charge to all age groups (1). According to the data in the WHO World Malaria Reports (1,2,6-15), the number of LLIN delivered by manufacturers relative to the population at risk was an average of 3.9% from 2010 to 2019. Assuming all LLINs last 3 years, this would be enough to cover 8.5% of the 62.8 million people at risk in 2019 (assuming an average of 2 people sleeping under each long-lasting insecticidal net). When it comes to IRS coverage and use at the population level, the coverage achieved by the NMCEP was 731 thousand people in 2019, representing 1.2% of the population at risk. The operational IRS coverage (relative to the population at risk) from 2010 to 2019 was an average of 1.7% (0.8% to 2.7%). IRS implementation has not been expanding, and the percentage of the population protected tends to be small. Regardless, the less extensive use of IRS vector control may reflect the more focal nature of malaria in the Philippines. Another reason for the low mortality rates could be due to the Philippines adopting the WHO artemisinin-based combination therapies (ACT) policy for case management and treatment of uncomplicated P. falciparum (1,8). As stated earlier, before treatment, the suspected malaria is confirmed by light microscopy, or RDTs, to distinguish malaria from non-malaria febrile illnesses. With all things considered, all these policies and interventions might have been enough to cure Plasmodium infection, thus preventing the progression of uncomplicated malaria to severe disease and limiting the transmission of malaria, eventually reducing morbidity and mortality.
Implications and actions needed
As previously shown by the WHO data, the Philippines malaria morbidity rates, prevalence, and transmission have been significantly reduced, and the country is now gearing towards malaria elimination. This could be due to the country prioritizing the prevention of infection, case management, and drug treatment. However, to verify a successful elimination program, diagnostic capabilities must also be adjusted to allow early detection of a malaria resurgence. Therefore, facilities and health workers capable of advanced diagnostic testing (e.g., PCR and LAMP) in malaria-endemic areas should be installed to ensure effective surveillance, detection, and immediate response to residual malaria transmission and outbreaks. In addition, continuous studies should also be conducted covering the current entomologic situation and the threats to success, such as the COVID-19 pandemic, that may hamper the delivery of malaria control and elimination related services.
Conclusions
Despite the technological advances in the commercially available malaria diagnostic tests (e.g., hematology analyzers, magneto-optic devices, PCR, LAMP, etc.), the diagnostic test applied in the Philippines seems to have not evolved much through the decades as it relies mostly on microscopy and RDTs. Yet the country has recorded a significant decrease in the number of confirmed cases and reported deaths from malaria since the year 2000, as observed by the NMCEP. Nevertheless, the diagnostic practice using microscopy and RDTs that was used to confirm cases may not provide an accurate picture of malaria incidence in a country gearing towards malaria elimination, as this might neglect light parasitemia and residual malaria transmission.
Acknowledgments
We would like to thank our advisers from the Department of Medical Technology, Faculty of Pharmacy, University of Santo Tomas for their guidance and support to complete this review.
Funding: None.
Footnote
Reporting Checklist: The authors have completed the PRISMA reporting checklist. Available at https://jphe.amegroups.com/article/view/10.21037/jphe-23-46/rc
Peer Review File: Available at https://jphe.amegroups.com/article/view/10.21037/jphe-23-46/prf
Conflicts of Interest: Both authors have completed the ICMJE uniform disclosure form (available at https://jphe.amegroups.com/article/view/10.21037/jphe-23-46/coif). The authors have no conflicts of interest to declare.
Ethical Statement: The authors are accountable for all aspects of the work in ensuring that questions related to the accuracy or integrity of any part of the work are appropriately investigated and resolved.
Open Access Statement: This is an Open Access article distributed in accordance with the Creative Commons Attribution-NonCommercial-NoDerivs 4.0 International License (CC BY-NC-ND 4.0), which permits the non-commercial replication and distribution of the article with the strict proviso that no changes or edits are made and the original work is properly cited (including links to both the formal publication through the relevant DOI and the license). See: https://creativecommons.org/licenses/by-nc-nd/4.0/.
References
- World Health Organization. World Malaria Report 2020. Geneva: World Health Organization; 2020.
- World Health Organization. World Malaria Report 2021. Geneva: World Health Organization; 2021.
- Al-Awadhi M, Ahmad S, Iqbal J. Current Status and the Epidemiology of Malaria in the Middle East Region and Beyond. Microorganisms 2021;9:338. [Crossref] [PubMed]
- Reyes RA, Fornace KM, Macalinao MLM, et al. Enhanced Health Facility Surveys to Support Malaria Control and Elimination across Different Transmission Settings in the Philippines. Am J Trop Med Hyg 2021;104:968-78. [Crossref] [PubMed]
- Dacuma MGB, Dimalibot JC, Baril JA, et al. Subpatent Plasmodium with mutant pfmdr1, pfcrt, and pvmdr1 alleles from endemic provinces in Mindanao, the Philippines: implications for local malaria elimination. Int J Infect Dis 2021;110:45-53. [Crossref] [PubMed]
- World Health Organization. World Malaria Report 2010. Geneva: World Health Organization; 2010.
- World Health Organization. World Malaria Report 2011. Geneva: World Health Organization; 2011.
- World Health Organization. World Malaria Report 2012. Geneva: World Health Organization; 2012.
- World Health Organization. World Malaria Report 2013. Geneva: World Health Organization; 2013.
- World Health Organization. World Malaria Report 2014. Geneva: World Health Organization; 2014.
- World Health Organization. World Malaria Report 2015. Geneva: World Health Organization; 2015.
- World Health Organization. World Malaria Report 2016. Geneva: World Health Organization; 2016.
- World Health Organization. World Malaria Report 2017. Geneva: World Health Organization; 2017.
- World Health Organization. World Malaria Report 2018. Geneva: World Health Organization; 2018.
- World Health Organization. World Malaria Report 2019. Geneva: World Health Organization; 2019.
- Spielman A, Perrone JB, Teklehaimanot A, et al. Malaria diagnosis by direct observation of centrifuged samples of blood. Am J Trop Med Hyg 1988;39:337-42. [Crossref] [PubMed]
- Rickman LS, Long GW, Oberst R, et al. Rapid diagnosis of malaria by acridine orange staining of centrifuged parasites. Lancet 1989;1:68-71. [Crossref] [PubMed]
- Kawamoto. Microscopy With Light Microscope and Interference. Lancet 1991;337:200-2. [Crossref]
- Gay F, Traoré B, Zanoni J, et al. Direct acridine orange fluorescence examination of blood slides compared to current techniques for malaria diagnosis. Trans R Soc Trop Med Hyg 1996;90:516-8. [Crossref] [PubMed]
- Post A, Kaboré B, Reuling IJ, et al. The XN-30 hematology analyzer for rapid sensitive detection of malaria: a diagnostic accuracy study. BMC Med 2019;17:103. [Crossref] [PubMed]
- Parra ME, Evans CB, Taylor DW. Identification of Plasmodium falciparum histidine-rich protein 2 in the plasma of humans with malaria. J Clin Microbiol 1991;29:1629-34. [Crossref] [PubMed]
- Shiff CJ, Premji Z, Minjas JN. The rapid manual parasight®-f test. a new diagnostic tool for Plasmodium falciparum infection. Trans R Soc Trop Med Hyg 1993;87:646-8. [Crossref] [PubMed]
- Banchongaksorn T, Yomokgul P, Panyim S, et al. A field trial of the ParaSight®-F test for the diagnosis of Plasmodium falciparum infection. Trans R Soc Trop Med Hyg 1996;90:244-5. [Crossref] [PubMed]
- Gaye O, Diouf M, Dansokho E, et al. Diagno is of Plasmodium falciparum malaria using ParaS ight F®, ICT malaria PF® and Malaria IgG CELISA® assays. Parasite 1998;5:189-92. [Crossref] [PubMed]
- Faiz A, Rashid R, Palit R. ParaSight-F test results in cerebral malaria patients before and after treatment in Chittagong Medical College Hospital, Bangladesh. Trans R Soc Trop Med Hyg 2000;94:56-7. [Crossref] [PubMed]
- Reyburn H, Mbakilwa H, Mwangi R, et al. Rapid diagnostic tests compared with malaria microscopy for guiding outpatient treatment of febrile illness in Tanzania: randomised trial. BMJ 2007;334:403. [Crossref] [PubMed]
- Neumann CG, Bwibo NO, Siekmann JH, et al. Comparison of blood smear microscopy to a rapid diagnostic test for in-vitro testing for r falciparum malaria in kenyan school children. East Afr Med J 2008;85:544-9. [PubMed]
- Falade CO, Ajayi IO, Nsungwa-Sabiiti J, et al. Malaria Rapid Diagnostic Tests and Malaria Microscopy for Guiding Malaria Treatment of Uncomplicated Fevers in Nigeria and Prereferral Cases in 3 African Countries. Clin Infect Dis 2016;63:S290-7. [Crossref] [PubMed]
- Ruizendaal E, Schallig HDFH, Scott S, et al. Evaluation of Malaria Screening during Pregnancy with Rapid Diagnostic Tests Performed by Community Health Workers in Burkina Faso. Am J Trop Med Hyg 2017;97:1190-7. [Crossref] [PubMed]
- Anabire NG, Aryee PA, Abdul-Karim A, et al. Prevalence of malaria and hepatitis B among pregnant women in Northern Ghana: Comparing RDTs with PCR. PLoS One 2019;14:e0210365. [Crossref] [PubMed]
- Makler MT, Hinrichs DJ. Measurement of the lactate dehydrogenase activity of Plasmodium falciparum as an assessment of parasitemia. Am J Trop Med Hyg 1993;48:205-10. [Crossref] [PubMed]
- Knobloch J, Henk M. Screening for malaria by determination of parasite-specific lactate dehydrogenase. Trans R Soc Trop Med Hyg 1995;89:269-70. [Crossref] [PubMed]
- Craig MH, Bredenkamp BL, Williams CHV, et al. Field and laboratory comparative evaluation of ten rapid malaria diagnostic tests. Transactions of the Royal Society of Tropical Medicine and Hygiene 2002;96:258-65. [Crossref] [PubMed]
- Mueller I, Betuela I, Ginny M, et al. The sensitivity of the OptiMAL rapid diagnostic test to the presence of Plasmodium falciparum gametocytes compromises its ability to monitor treatment outcomes in an area of Papua New Guinea in which malaria is endemic. J Clin Microbiol 2007;45:627-30. [Crossref] [PubMed]
- Belizario VY, Pasay CJ, Bersabe MJ, et al. Field evaluation of malaria rapid diagnostic tests for the diagnosis of P. falcifarum and non P. falcifarum infections. Southeast Asian J Trop Med Public Health 2005;36:552-61. [PubMed]
- Jamjoom GA. Dark-field microscopy for detection of malaria in unstained blood films. J Clin Microbiol 1983;17:717-21. [Crossref] [PubMed]
- Delahunt C, Horning MP, Wilson BK, et al. Limitations of haemozoin-based diagnosis of Plasmodium falciparum using dark-field microscopy. Malar J 2014;13:147. [Crossref] [PubMed]
- Scott CS, van Zyl D, Ho E, et al. Automated detection of malaria-associated intraleucocytic haemozoin by Cell-Dyn CD4000 depolarization analysis. Clin Lab Haematol 2003;25:77-86. [Crossref] [PubMed]
- Newman DM, Heptinstall J, Matelon RJ, et al. A magneto-optic route toward the in vivo diagnosis of malaria: preliminary results and preclinical trial data. Biophys J 2008;95:994-1000. [Crossref] [PubMed]
- Omucheni DL, Kaduki KA, Bulimo WD, et al. Application of principal component analysis to multispectral-multimodal optical image analysis for malaria diagnostics. Malar J 2014;13:485. [Crossref] [PubMed]
- de Melo GC, Netto RLA, Mwangi VI, et al. Performance of a sensitive haemozoin‐based malaria diagnostic test validated for vivax malaria diagnosis in Brazilian Amazon. Malar J 2021;20:146. [Crossref] [PubMed]
- Franzén L, Shabo R, Perlmann H, et al. Analysis of clinical specimens by hybridization with probe containing repetitive DNA from Plasmodium falcifarum. Lancet 1984;323:525-8. [Crossref] [PubMed]
- Mclaughlin GL, Ruth JL, Jablonski E, et al. Use of enzyme-linked synthetic DNA in diagnosis of falcifarum malaria. Lancet 1987;1:714-6. [Crossref] [PubMed]
- Jaureguiberry G, Hatin I, d'Auriol L, et al. PCR detection of Plasmodium falciparum by oligonucleotide probes. Mol Cell Probes 1990;4:409-14. [Crossref] [PubMed]
- Tirasophon W, Ponglikitmongkol M, Wilairat P, et al. A novel detection of a single Plasmodium falcifarum in infected blood. Biochem Biophys Res Commun 1991. Available online: https://builtsurvey.utm.my/
- Snounou G, Viriyakosol S, Zhu XP, et al. High sensitivity of detection of human malaria parasites by the use of nested polymerase chain reaction. Mol Biochem Parasitol 1993;61:315-20. [Crossref] [PubMed]
- Snounou G, Viriyakosol S, Jarra W, et al. Identification of the four human malaria parasite species in field samples by the polymerase chain reaction and detection of a high prevalence of mixed infections. Mol Biochem Parasitol 1993;58:283-92. [Crossref] [PubMed]
- Laoboonchai A, Kawamoto F, Thanoosingha N, et al. PCR-based ELISA technique for malaria diagnosis of specimens from Thailand. Trop Med Int Health 2001;6:458-62. [Crossref] [PubMed]
- Chishimba S, Mwenda M, Mambwe B, et al. Prevalence of Plasmodium falciparum and Non-falciparum Infections by Photo-Induced Electron Transfer-PCR in a Longitudinal Cohort of Individuals Enrolled in a Mass Drug Administration Trial in Southern Province, Zambia. Am J Trop Med Hyg 2020;103:82-9. [Crossref] [PubMed]
- Gadalla AAH, Siciliano G, Farid R, et al. Real-time PCR assays for detection and quantification of early P. falciparum gametocyte stages. Sci Rep 2021;11:19118. [Crossref] [PubMed]
- Nuin NA, Tan AF, Lew YL, et al. Comparative evaluation of two commercial real-time PCR kits (QuantiFastTM and abTESTM) for the detection of Plasmodium knowlesi and other Plasmodium species in Sabah, Malaysia. Malar J 2020;19:306. [Crossref] [PubMed]
- Poon LLM, Wong B, Ma E. Sensitive and Inexpensive Molecular Test for Falcipa- rum Malaria: Detecting Plasmodium falciparum DNA Directly from Heat-Treated Blood by Loop-Mediated Isothermal Amplification. Clin Chem 2006;52:303-6. [Crossref] [PubMed]
- Han ET, Watanabe R, Sattabongkot J, et al. Detection of four Plasmodium species by genus- and species-specific loop-mediated isothermal amplification for clinical diagnosis. J Clin Microbiol 2007;45:2521-8. [Crossref] [PubMed]
- Lucchi NW, Gaye M, Diallo MA, et al. Evaluation of the Illumigene Malaria LAMP: A Robust Molecular Diagnostic Tool for Malaria Parasites. Sci Rep 2016;6:36808. [Crossref] [PubMed]
- Tegegne B, Getie S, Lemma W, et al. Performance of loop-mediated isothermal amplification (LAMP) for the diagnosis of malaria among malaria suspected pregnant women in Northwest Ethiopia. Malar J 2017;16:34. [Crossref] [PubMed]
- Kemleu SGZT, Ngando L, Nguekeng E, et al. Diagnostic performance of a rapid whole blood-based RT-LAMP method for malaria diagnosis among apparently healthy blood donors and febrile neonates in Cameroon. PLoS One 2021;16:e0246205. [Crossref] [PubMed]
- Cordray MS, Richards-Kortum RR. Emerging nucleic acid-based tests for point-of-care detection of malaria. Am J Trop Med Hyg 2012;87:223-30. [Crossref] [PubMed]
- Danwang C, Kirakoya-Samadoulougou F, Samadoulougou S. Assessing field performance of ultrasensitive rapid diagnostic tests for malaria: a systematic review and meta-analysis. Malar J 2021;20:245. [Crossref] [PubMed]
- Erdman LK, Kain KC. Molecular diagnostic and surveillance tools for global malaria control. Travel Med Infect Dis 2008;6:82-99. [Crossref] [PubMed]
- Feleke DG, Alemu Y, Yemanebirhane N. Performance of rapid diagnostic tests, microscopy, loop-mediated isothermal amplification (LAMP) and PCR for malaria diagnosis in Ethiopia: a systematic review and meta-analysis. Malar J 2021;20:384. [Crossref] [PubMed]
- Hawkes M, Kain KC. Advances in malaria diagnosis. Expert Rev Anti Infect Ther 2007;5:485-95. [Crossref] [PubMed]
- Jain P, Chakma B, Patra S, et al. Potential biomarkers and their applications for rapid and reliable detection of malaria. Biomed Res Int 2014;2014:852645. [Crossref] [PubMed]
- Kasetsirikul S, Buranapong J, Srituravanich W, et al. The development of malaria diagnostic techniques: a review of the approaches with focus on dielectrophoretic and magnetophoretic methods. Malar J 2016;15:358. [Crossref] [PubMed]
- Krampa FD, Aniweh Y, Awandare GA, et al. Recent Progress in the Development of Diagnostic Tests for Malaria. Diagnostics (Basel) 2017;7:54. [Crossref] [PubMed]
- Han ET. Loop-mediated isothermal amplification test for the molecular diagnosis of malaria. Expert Rev Mol Diagn 2013;13:205-18. [Crossref] [PubMed]
- Yerlikaya S, Owusu EDA, Frimpong A, et al. A Dual, Systematic Approach to Malaria Diagnostic Biomarker Discovery. Clin Infect Dis 2022;74:40-51. [Crossref] [PubMed]
- Pollak JJ, Houri-Yafin A, Salpeter SJ. Computer Vision Malaria Diagnostic Systems—Progress and Prospects. Front Public Health 2017;5:219. [Crossref] [PubMed]
- Poostchi M, Silamut K, Maude RJ, et al. Image analysis and machine learning for detecting malaria. Transl Res 2018;194:36-55. [Crossref] [PubMed]
- Kolluri N, Klapperich CM, Cabodi M. Towards lab-on-a-chip diagnostics for malaria elimination. Lab Chip 2017;18:75-94. [Crossref] [PubMed]
- Fitri LE, Widaningrum T, Endharti AT, et al. Malaria diagnostic update: From conventional to advanced method. J Clin Lab Anal 2022;36:e24314. [Crossref] [PubMed]
- Fontecha G, Mejía RE, Banegas E, et al. Deletions of pfhrp2 and pfhrp3 genes of Plasmodium falciparum from Honduras, Guatemala and Nicaragua. Malar J 2018;17:320. [Crossref] [PubMed]
- Fung AO, Damoiseaux R, Grundeen S, et al. Quantitative detection of PfHRP2 in saliva of malaria patients in the Philippines. Malar J 2012;11:175. [Crossref] [PubMed]
- Bell D, Go R, Miguel C, et al. Diagnosis of malaria in a remote area of the Philippines: comparison of techniques and their acceptance by health workers and the community. Bull World Health Organ 2001;79:933-41. [PubMed]
- Luchavez J, Lintag ME, Coll-Black M, et al. An assessment of various blood collection and transfer methods used for malaria rapid diagnostic tests. Malar J 2007;6:149. [Crossref] [PubMed]
- Foley DH, Torres EP, Mueller I, et al. Host-dependent Anopheles flavirostris larval distribution reinforces the risk of malaria near water. Trans R Soc Trop Med Hyg 2003;97:283-7. [Crossref] [PubMed]
- Luchavez J, Baker J, Alcantara S, et al. Laboratory demonstration of a prozone-like effect in HRP2-detecting malaria rapid diagnostic tests: implications for clinical management. Malar J 2011;10:286. [Crossref] [PubMed]
- Matsumoto-Takahashi EL, Tongol-Rivera P, Villacorte EA, et al. Determining the active role of microscopists in community awareness-raising activities for malaria prevention: a cross-sectional study in Palawan, the Philippines. Malar J 2013;12:384. [Crossref] [PubMed]
- Roth JM, Korevaar DA, Leeflang MM, et al. Molecular malaria diagnostics: A systematic review and meta-analysis. Crit Rev Clin Lab Sci 2016;53:87-105. [Crossref] [PubMed]
- World Health Organization. Response plan to pfhrp2 gene. Geneva: World Health Organization; 2019.
Cite this article as: Palmares AJ, Martin I G. The development of commercially available malaria diagnostic tests and their extent of application in the Philippines: a systematic review. J Public Health Emerg 2023;7:30.